Gearing up for the Clinic: Unlocking the Mysteries of RNA Editing
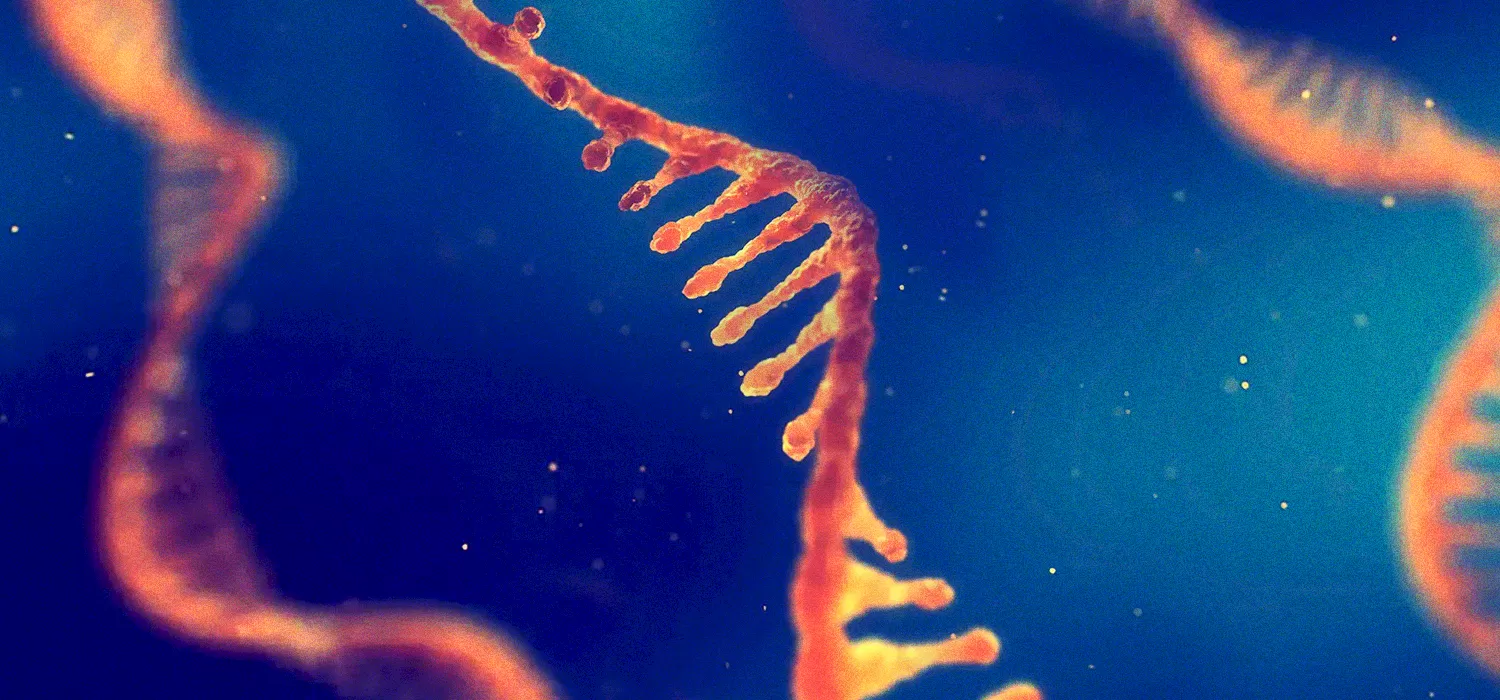
In the realm of molecular biology, where the essence of life unfolds at the sub-microscopic level, RNA editing stands as a remarkable phenomenon. While DNA is often hailed as the blueprint of life, RNA editing provides a mechanism where the genetic code can be altered without affecting the original DNA blueprint.
This blog post explores the intricacies of RNA editing, unraveling its significance and implications in the scheme of biology, and updates the Rett community on important research moving closer to the clinic.
Understanding RNA Editing
So, what are the first principles we can learn about RNA editing? At some point in biology class the central dogma was discussed – that DNA makes an RNA copy (transcription) and that RNA then is read to make protein (translation). All of these molecules are in every cell and are responsible for the natural differences found between muscle cells and blood cells and brain cells, etc. DNA is a molecule composed of two intertwined strands like a zipper. Genes on these DNA strands are used to make an RNA copy, which is then used by the cell to produce that gene’s protein.
At its core, RNA editing refers to the process by which the copied RNA bases are changed, leading to alterations in the final protein-coding sequence. Unlike DNA editing, which involves changes at the genomic level and are permanent, RNA editing occurs in the sea of RNA copies, also known as the transcriptome, the entire set of RNA molecules produced in a cell. The RNA editing process can result in the insertion, deletion, or modification of bases within RNA molecules, giving rise to diverse but related RNAs that have distinct functions.
Mechanisms of RNA Editing
One of the most well-studied forms of RNA editing is adenosine-to-inosine (A-to-I) editing, performed by adenosine deaminases (ADAR) acting on RNA. ADARs convert adenosine (A) bases into inosine (I), which is interpreted as guanosine (G) during translation of mRNA to make protein. This type of editing has the ability to alter mutations in the RNA strand as copied from the DNA, back to the correct base, as if there was no mutation in the DNA to begin with.
Biological Significance of RNA Editing
The significance of RNA editing impacts many aspects of cellular function and development. By changing the transcriptome with purposeful edits, RNA editing creates protein complexity, allowing cells to fine-tune gene expression and adapt to changing environmental conditions. In the nervous system, RNA editing plays a critical role, shaping brain cell function and interactions. Abnormal RNA editing in the brain has been linked to neurological disorders, including epilepsy, schizophrenia, and Alzheimer's disease, underscoring its importance in maintaining proper neuronal function.
RNA Editing and Rett
RSRT has gotten important results from its initial investment in the lab of Dr. Peter Beal, UC Davis. Dr. Beal is an international authority in the mechanisms of RNA editing and the rules needed to design effective small RNA molecules to carry out RNA editing in vivo.
Dr Beal’s work on RNA editing includes a focus on Rett syndrome, where his work on multiple mutations in has led to partnership with ProQR, a Dutch biotechnology company developing small therapeutic RNAs. ProQR and RSRT have recently announced an agreement to develop small RNAs for treatment of multiple Rett mutations. Small RNAs were pioneered by small antisense oligonucleotides (ASO), like Spinraza for Spinal Muscular Atrophy (SMA). Due to direct delivery without requiring a viral or non-viral delivery platform, and prior success in drug development, this approach can proceed more rapidly through clinical development than more novel DNA based gene corrections.
One of the key breakthroughs in Beal’s research was understanding how ADAR performs a targeted A-I change in the RNA molecule and thereby gaining the ability to direct ADAR to a specific mutation of interest. The approach is broad and can be directed to a large number of Rett mutations.
In addition to his scientific achievements, Peter Beal is renowned for his mentorship and commitment to scientific education. As a professor at the University of California, Davis, he has inspired many students and researchers to explore the fascinating realm of RNA biology, and applied his work towards a cure for Rett. As we continue to unravel the complexities of RNA editing we are indebted to visionaries like Peter Beal who have dedicated their careers to unraveling the secrets of the RNA world.
Future Directions and Challenges
As our understanding of RNA editing continues to evolve, supported by significant advances in innovative technologies, questions and challenges remain. Unraveling RNA editing mechanisms, identifying novel editing events, and deciphering their consequences represent ongoing areas of research.
The FDA and other regulatory agencies are also working to accelerate testing of genetic treatments in rare disease. Therapeutics like RNA editing are advantageous to pursue in Rett because they are platform technologies, which means once the first RNA mutation is successfully corrected, the tools to direct ADAR’s attention and correct other mutations can rapidly be swapped in, shaving off vast amounts of time and research that are typically needed to develop separate therapeutics.
Conclusion
Delving deeper into the mysteries of RNA editing unlocks new insights into the complexity of gene regulation and opens doors to discoveries that may be transformative for the future of medicine. In the dance to correct or replace mutations, RNA editing emerges as an intriguing choreographer, orchestrating dynamic changes within the transcriptome with precision and rivaling approaches for DNA editing or gene replacement. Compared to gene replacement approaches that add in a healthy copy of MECP2, RNA editing corrects the protein deficiency in place and maintains normal cellular signals guiding MECP2 expression.
The greatest challenge to overcome in gene therapies and correction in the brain is delivery. Current gene therapy clinical studies utilize AAV, a viral based delivery platform to deliver healthy copies of MECP2 to brain cells. This adds high cost to treatments, and can be toxic at high doses, and due to immune issues is limited for now to only one treatment per patient. Small RNAs, on the other hand, can be delivered without being packaged, and with some modifications to improve stability, can be active for 6 – 9 months. That makes potential re-dosing schedules very reasonable for patients.
RSRT is very excited about the horizon we can see for the next 2 – 3 years for RNA editing, and supporting both research and industry development to achieve new treatment opportunities in RTT.